Reversing The EVM: Raw Calldata
8 min read
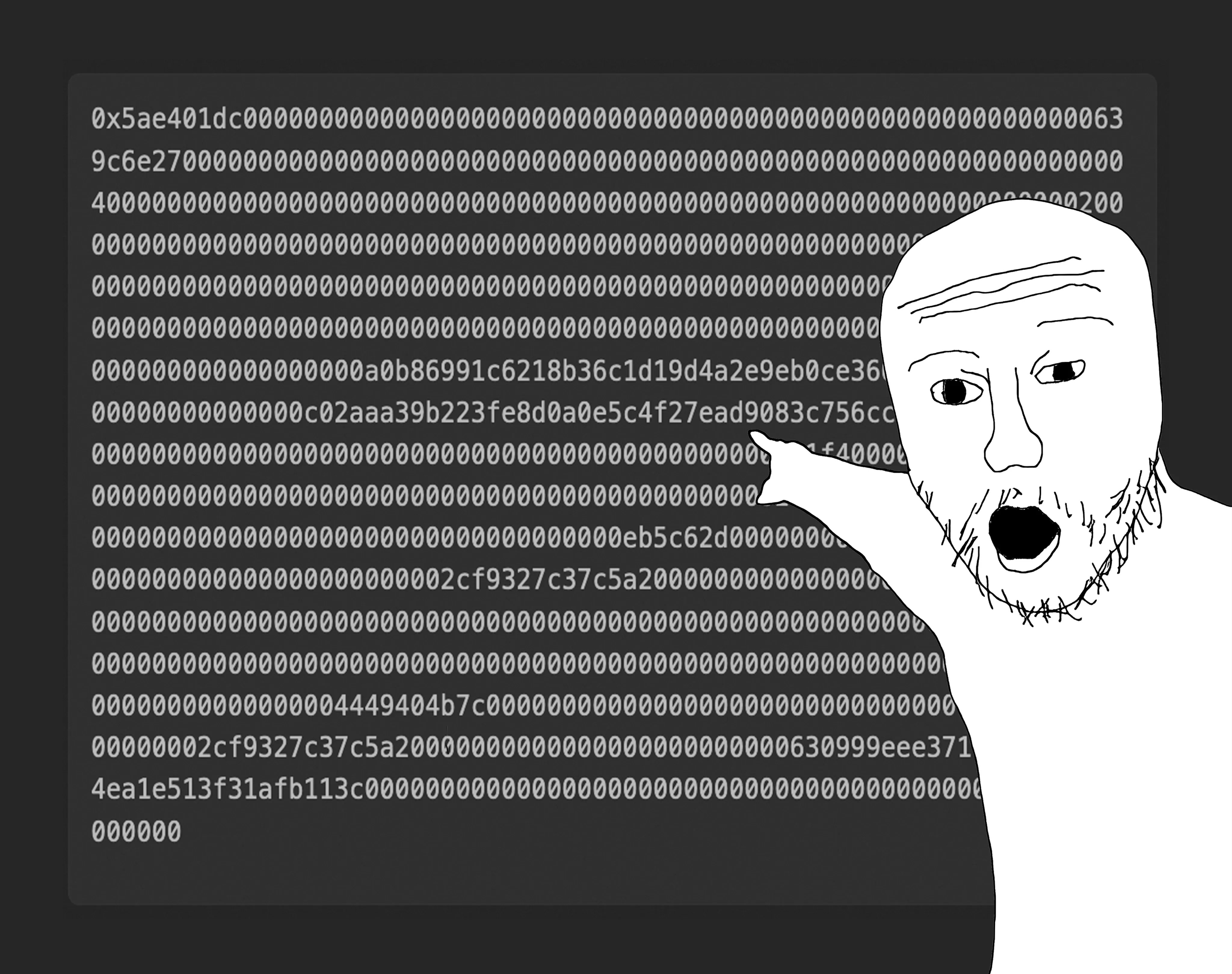
You may have have wondered how to decipher and read evm calldata, then attempted to read the transaction calldata of an Ethereum smart contract, only to become confused at a certain point. The EVM (and other L1 forks) encode and decode calldata in a specific way for static and dynamic types, which can be initially confusing. In this article, we will delve into the encoding sequence of calldata so that you can comprehend any verified or unverified smart contract transactions and understand the bytes. By doing so, I hope to empower you to create your own raw calldata.
What Is Calldata?
Calldata is the encoded parameters that we send to functions, in this case to smart contracts on the Ethereum Virtual Machine (EVM). Each piece of calldata is 32 bytes long, or 64 characters. There are two types of calldata: static and dynamic.
Static variables are fairly straightforward to understand. Dynamic variables, on the other hand, are much more complex, and this is likely the reason why you may have difficulty reading raw calldata intuitively. However, once we go through how dynamic variables work, you will be able to read raw calldata with ease.
To begin, let’s understand how calldata is encoded and decoded to establish a foundation of how it all works.
Encoding Calldata
To encode types, you can pass them into the `abi.encode(parameters)“ method to generate raw calldata.
If you want to encode calldata for a specific interfaced function, you can use abi.encodeWithSelector(selector, parameters). This will be the same as passing in the function and it’s parameters directly.
For example:
interface A { function transfer(uint256[] memory ids, address to) virtual external;}
contract B { function a(uint256[] memory ids, address to) external pure returns(bytes memory) { return abi.encodeWithSelector(A.transfer.selector, ids, to); }}
The method .selector
generates the 4-bytes that represents that method on the interface. We use it
to tell the EVM that we’re sending our calldata to that function. This is how UniswapV2 enables
flashswaps!
There is also abi.encodePacked(...)
which is efficiently put all dynamic variables with eachother,
removing the 0 padding. The problem with it is that it doens’t prevent collisions and should only be
used when you know for certain the types and lengths of the parameters.
Decoding calldata
So you have your calldata, how do you decode it?
If the calldata was created with abi.encode(...)
then we can decode the parameters with
abi.decode(...)
by passing in the parameters we want to decode the calldata into.
For example:
(uint256 a, uint256 b) = abi.decode(data, (uint256, uint256))
Where data
represents the calldata being passed in.
Now that we understand how to encode and decode parameters, we can move onto the different variable types and how they are reflected in the calldata output.
Static Variables
Static variables are simply the encoded representation of the following types: uint
s, int
s,
address
, bool
, bytes1
to bytes32
(including function selector), and tuple
s (however they
can have dynamic variables in them).
For example, lets say we’re interacting with the following contract:
pragma solidity 0.8.17;contract Example { function transfer(uint256 amount, address to) external;}
With the input parameters:
amount: 1300655506address: 0x68b3465833fb72A70ecDF485E0e4C7bD8665Fc45
We would generate the calldata:
0x000000000000000000000000000000000000000000000000000000004d866d9200000000000000000000000068b3465833fb72a70ecdf485e0e4c7bd8665fc45
But… how tf do we read this?
Well, lets chop it up into readable pieces, by first removing the prefix 0x
and then breaking each
line into 64 character (or 32 byte) parts
0x// uint256000000000000000000000000000000000000000000000000000000004d866d92// address00000000000000000000000068b3465833fb72a70ecdf485e0e4c7bd8665fc45
Cool, now we know the first 32-bytes is the uint256 amount
variable and the 2nd 32-bytes is the
address to
one.
Functions
But what if we wanted to call the transfer
function directly?
We would need to know what parameters types takes in order and use a hashing mechanism called
keccak256
which turns the inputted data into a 32-byte hash.
In this case, to hash:
function transfer(uint256 amount, address to) external;
We would do:
keccak256("transfer(uint256,address)");
Which would return the following 32-byte hash:
0xb7760c8fd605b6ef5a068e1720c115665f9699a5c439e3c0ee9709290ff8a3bb
To get the function signature we only need the first 4-bytes (or 8 characters, excluding the 0x
prefix), b7760c8f
.
This 4-byte signature, b7760c8f
, is how we tell the EVM that we’re interacting with that function
and the following calldata is being passed in as parameters.
For example, if we were to call transfer
with the same parameters as before in # Static Variables
we would grab the existing calldata:
0x000000000000000000000000000000000000000000000000000000004d866d9200000000000000000000000068b3465833fb72a70ecdf485e0e4c7bd8665fc45
And add b7760c8f
to the start of the first 4 bytes of the first 32-bytes:
0xb7760c8f000000000000000000000000000000000000000000000000000000004d866d9200000000000000000000000068b3465833fb72a70ecdf485e0e4c7bd8665fc45
or
0xb7760c8f000000000000000000000000000000000000000000000000000000004d866d9200000000000000000000000068b3465833fb72a70ecdf485e0e4c7bd8665fc45
You may be wondering, how is the calldata parameter actually inputted into the function with the embedded signature?
The answer is, the contract’s bytecode reads it by targetting the function b7760c8f
, then
replacing it with 00000000
padding then passing in the parameter.
Dynamic Variables
Dynamic variables are non-fixed-size types, including bytes
, string
, and dynamic arrays <T>[]
,
as well as fixed arrays <T>[N]
.
The structure of dynamic types always starts with an offset, which is the hexadecimal representation
of where the dynamic type begins. For example, a hex of 20
represents 32-bytes
. Once we reach
the offset, there is a smaller number that represents the length of the type.
Tldr; 1st 32-bytes = offset, 2nd 32-bytes = length, the rest are elements.
For arrays, this length represents the number of elements contained in the array. For bytes and
string types, it represents the length of the type. For example, the string "Hello World!"
is
12-bytes long, with each character being 1-byte. Keep in mind that these types start on the
left-hand side of the calldata, rather than the right-hand side like everything else.
For example, here’s the string
"Hello World!"
encoded:
0x0000000000000000000000000000000000000000000000000000000000000020000000000000000000000000000000000000000000000000000000000000000c48656c6c6f20576f726c64210000000000000000000000000000000000000000
Observe how the first 32-bytes represents the hexadecimal offset of 20
, which is 32
in decimal.
So we skip 32-bytes from the start of
0000000000000000000000000000000000000000000000000000000000000020
, bringing us to the next line
with a hex of 0c
, a decimal of 12
, representing length of our string
in bytes. Now when we
convert 48656c6c6f20576f726c6421
to a string
type it returns our original value.
Congrats! Now you know how to read dynamic types.
Decoding Static And Dynamic Parameters
Lets say we’re interacting with the following contract:
pragma solidity 0.8.17;contract Example { function transfer(uint256[] memory ids, address to) external;}
With the following parameters for transfer
:
ids: ["1234", "4567", "8910"]to: 0xf8e81D47203A594245E36C48e151709F0C19fBe8
We would generate the calldata:
0x8229ffb60000000000000000000000000000000000000000000000000000000000000040000000000000000000000000f8e81d47203a594245e36c48e151709f0c19fbe8000000000000000000000000000000000000000000000000000000000000000300000000000000000000000000000000000000000000000000000000000004d200000000000000000000000000000000000000000000000000000000000011d700000000000000000000000000000000000000000000000000000000000022ce
We can chop this up into a more readable form:
// prefix we discard0x// fn selector we're calling (`transfer(uint[], address)`)8229ffb6// `uint256[] ids` param array offset (64-bytes below from start of this line)0000000000000000000000000000000000000000000000000000000000000040// `address to` param000000000000000000000000f8e81d47203a594245e36c48e151709f0c19fbe8// length of `ids` array; 3 inputs0000000000000000000000000000000000000000000000000000000000000003// 1st `ids` param00000000000000000000000000000000000000000000000000000000000004d2// 2nd `ids` param00000000000000000000000000000000000000000000000000000000000011d7// 3rd `ids` param00000000000000000000000000000000000000000000000000000000000022ce
Notice how the array parameter is represented by an offset to where the array begins. Then we move
onto the second param, the address
type, then finishing off the array type.
Now that we know how to read both static + dynamic parameters, let’s dissect a more complex example!
Decoding A Multicall’s Calldata
We’re going to be a UniswapV3 multicall’s input calldata from this transaction. Here the user calls 3 different functions from the multicall function.
Etherscan is nice enough to give us a simple decoded version:
MethodID: 0xac9650d8000000000000000000000000000000000000000000000000000000000000002000000000000000000000000000000000000000000000000000000000000000030000000000000000000000000000000000000000000000000000000000000060000000000000000000000000000000000000000000000000000000000000012000000000000000000000000000000000000000000000000000000000000002c0000000000000000000000000000000000000000000000000000000000000008413ead56200000000000000000000000061fe7a5257b963f231e1ef6e22cb3b4c6e28c531000000000000000000000000c02aaa39b223fe8d0a0e5c4f27ead9083c756cc20000000000000000000000000000000000000000000000000000000000002710000000000000000000000000000000000000000000831162ce86bc88052f80fd0000000000000000000000000000000000000000000000000000000000000000000000000000000000000000000000000000000000000000000001648831645600000000000000000000000061fe7a5257b963f231e1ef6e22cb3b4c6e28c531000000000000000000000000c02aaa39b223fe8d0a0e5c4f27ead9083c756cc20000000000000000000000000000000000000000000000000000000000002710fffffffffffffffffffffffffffffffffffffffffffffffffffffffffffaf178000000000000000000000000000000000000000000000000000000000000000000000000000000000000000000000000000000000002e3bdc25349196582d720000000000000000000000000000000000000000000000000c249fdd32778000000000000000000000000000000000000000000000002e1e525c2ef9dcec50c53000000000000000000000000000000000000000000000000c1cd7c9adfb0d9dc000000000000000000000000ed6c2cb9bf89a2d290e59025837454bf1f144c5000000000000000000000000000000000000000000000000000000000635ce8bf00000000000000000000000000000000000000000000000000000000000000000000000000000000000000000000000000000000000000000000000412210e8a00000000000000000000000000000000000000000000000000000000
We will modify this a bit and expand upon this line-by-line to make it even more readable. Keep in
mind, each value is in hexadecimal format and 20 hex == 32-bytes
for quick reference.
MethodID: 0xac9650d8// offset of array_1 (starting next line)0000000000000000000000000000000000000000000000000000000000000020// length of array_1 (how many elements in array)0000000000000000000000000000000000000000000000000000000000000003// offset of 1st element in array_1, array_1A (96-bytes / 32 = 3)0000000000000000000000000000000000000000000000000000000000000060// offset of 2nd element in array_1, array_1B (288-bytes / 32 = 9)0000000000000000000000000000000000000000000000000000000000000120// offset of 3rd element in array_1, array_1C (704-bytes / 32 = 22)00000000000000000000000000000000000000000000000000000000000002c0
// length 1st element of array_1, array_1A (132-bytes (inc. selector))0000000000000000000000000000000000000000000000000000000000000084
// here we'll read the next 132-bytes// fn selector; 4 of 13213ead562// 1st param; 36 of 13200000000000000000000000061fe7a5257b963f231e1ef6e22cb3b4c6e28c531// 2nd param; 68 of 132000000000000000000000000c02aaa39b223fe8d0a0e5c4f27ead9083c756cc2// 3rd param; 100 of 1320000000000000000000000000000000000000000000000000000000000002710// 4th param; 132 of 132// this marks the end of array_1A000000000000000000000000000000000000000000831162ce86bc88052f80fd
// 32-bytes of `0` indicating next elemet0000000000000000000000000000000000000000000000000000000000000000// length 2nd element of array_1, array_1B (356-bytes (inc. selector))// we have 4-bytes missing due to the embedded fn selector, 13ead562// the next fn selector, 88316456, will be inserted here00000000000000000000000000000000000000000000000000000164
// here we'll read the next 356-bytes// fn selector; 4 of 35688316456// 1st param; 36 of 35600000000000000000000000061fe7a5257b963f231e1ef6e22cb3b4c6e28c531// 2nd param; 68 of 356000000000000000000000000c02aaa39b223fe8d0a0e5c4f27ead9083c756cc2// 3rd param; 100 of 3560000000000000000000000000000000000000000000000000000000000002710// 4th param; 132 of 356// notice how all the `0`s are `f`s. this indicates a `int` type!fffffffffffffffffffffffffffffffffffffffffffffffffffffffffffaf178// 5th param; 164 of 356// we have 32-bytes of `0`, but since we're still reading the bytes// we know this is a paramter, representing 0 of a type0000000000000000000000000000000000000000000000000000000000000000// 6th param; 196 of 35600000000000000000000000000000000000000000002e3bdc25349196582d720// 7th param; 228 of 356000000000000000000000000000000000000000000000000c249fdd327780000// 8th param; 260 of 35600000000000000000000000000000000000000000002e1e525c2ef9dcec50c53// 9th param; 292 of 356000000000000000000000000000000000000000000000000c1cd7c9adfb0d9dc// 10th param; 324 of 356000000000000000000000000ed6c2cb9bf89a2d290e59025837454bf1f144c50// 11th param; 356 of 356// this marks the end of array_1B00000000000000000000000000000000000000000000000000000000635ce8bf
// 32-bytes of `0` indicating next elemet0000000000000000000000000000000000000000000000000000000000000000// this is the same thing as before, the length!// we can see there's only 32-bytes left so we can conclude// that it's going to be a fn with no inputs00000000000000000000000000000000000000000000000000000004
// a call to the fn selector 12210e8a; 4 of 412210e8a00000000000000000000000000000000000000000000000000000000
Now you’re able to read raw embedded dynamic types!
Final
I hope this information has helped you understand how calldata is encoded, decoded, and read. It took me some time to research and experiment with it all in order to learn, but it was worth it. The next step from here is to learn how to read bytecode in order to understand the EVM at its lowest level (Then everything becomes open-source >:D).
I appreciate you for taking the time to read this article. I hope you found value in this, anon!
Share this Article
Recent Articles
-
Generating Custom Assembly Smart Contracts
2 years ago I wrote a component that allowed me to generate any custom assembly smart contract on the fly to automatically create exploits without needing to do any manual work. I had a montiroing system that would provide the inputs and the bytecode generator would chug along and spit out an executable program that I could deploy and call on with a bundle of transactions. In this article I'll share the core of that codebase to get you up to speed! Buckle up, anon. There isn't any other article like this revealing these trade secrets!
-
Baiting MEV Bots: UniV2 Token Trapper
So many MEV bots take money from people but why don't people take money from them? I always thought about this when I was in my web3 cybersec assembly arc. I got quite fascinated with reverse engineering them and the contracts they interected with and realised there are some interesting things you can do with the uniswap code, since it has a few assumptions with the tokens you provide to create the pairs. Although not very practical it's definitely an intereting thought experiment that can provoke some further creativity!
-
Starting Malware Development
After spending years in MEV and web3 infosec DeGatchi explains his reasoning behind switching to malware development. Although seemingly less money and entering an alreaedy mature field, it's clearly the most powerful long term decision to be made -- especially when combining malware development with custom evolutionary AI algorithms.